Characterization of adsorption-based gas separation processes
Adsorption-based processes are believed to bear potential within the set of technologies featured in carbon dioxide capture, utilization, and storage (CCUS). The most common approach for post-combustion CO2 recovery exploits liquid phase absorption, where amine-based solvents or ammonia are employed to scrub CO2 from a flue gas stream. Adsorption-based processes have recently attracted attention due to the use of solid materials, to their non-volatility and to their possibly low energy consumption associated with the regeneration step.
At SPL we study and develop adsorption-based processes for the recovery of carbon dioxide from process or flue gases, while considering the presence of water vapor. Thereby two main research directions are considered: the characterization of potential adsorbents and the design and development of efficient process cycles, including an optimal regeneration strategy based on temperature and/or pressure swing adsorption.
Adsorption Equilibria
At the beginning of the development of an adsorption based separation process stands the screening and the characterization of promising candidate sorbent materials. Ideally, the sorbent features a large cyclic capacity, selectivity, stability and regenerability as well as fast kinetics. Adsorption equilibrium data over a range of pressure at different temperature levels, so called isotherms, provide information on the achievable cyclic capacity. Such data is obtained with a gravimetric method using a Magnetic Suspension Balance (MSB) by Rubotherm (see Figure 1). In addition to the adsorption equilibrium of single components, we also measure the adsorption equilibrium of mixtures of two gases, such as nitrogen and CO2, which provide information about the selectivity of the material for one or the other species, and allows us to choose an appropriate model to describe how they affect each other. An example of this binary adsorption is shown in Figure 2. This information gives a first indication of the suitability of a candidate material. In our lab, we have studied commercial materials such as activated carbon [1] and zeolites [8], but also novel materials such as metal-organic frameworks [3].
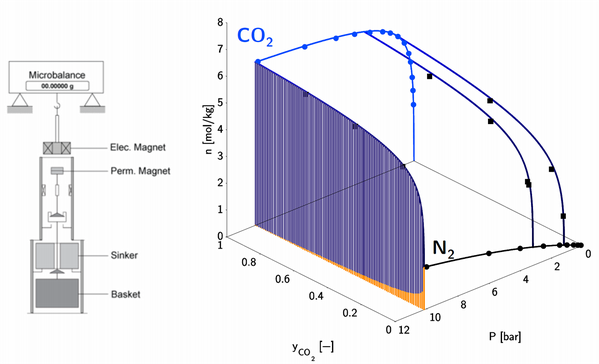
Adsorption Dynamics (Fixed Bed)
Dynamic measurements are carried out by performing breakthrough experiments using fixed-bed apparatus packed with sorbent material. The temperature is recorded at several places along the column, and the composition of the outflowing gas is measured on-line by a mass spectrometer. A thorough characterization of the adsorption kinetics and of the heat transport is being carried out for model systems including CO2, N2, H2 and H2O. Fitting of experimental data to a detailed mathematical model allow determining the heat and mass transport parameters and consolidating the equilibrium data [2,4,5,7]. More complex dynamics involved in systems which exhibit hysteresis, e.g. water on activated carbon, are also being studied with these tools.
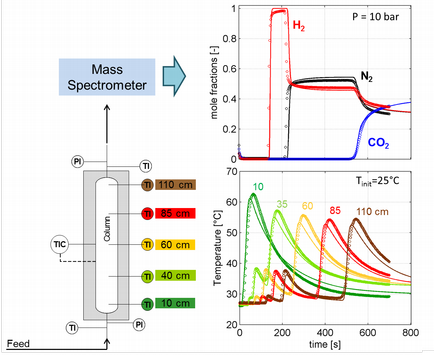
Process model validation (lab-scale plant)
Once the adsorption equilibrium and kinetics are characterized and can be described by model, this model can be used to describe the different steps that a column undergoes in a cyclic adsorption process. In the case of pressure swing adsorption (PSA), for example, these usually include:
Pressurization increasing the pressure inside the column to the adsorption pressure;
Adsorption feeding the mixture to be separated, and producing the light component (e.g. hydrogen) while the heavy component is; retained in the column;
Blowdown reducing the pressure inside the column to the desorption pressure;
Purge sweep the column using either the feed or the light product.
In addition, a recycle step such as a pressure equalization might be included. As these cover a wide range of conditions, it is important to validate the model for full cycles before using it for parametric studies or optimization. In order to do this, a two-column PSA plant (see Figure 5) is used to run cycles with different operating conditions, and testing the model’s predictions of key performance indicators such as the purity of the products and the recovery of the important components [6]. Testing this model rigorously is important for the model to be used for further process design.
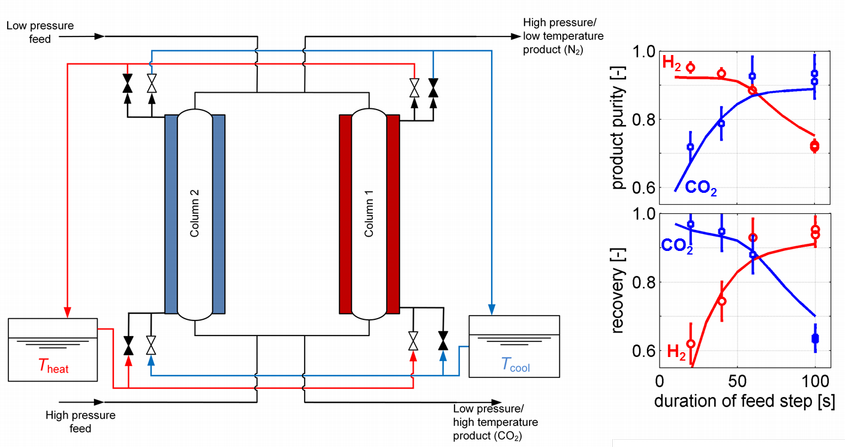
References
- external page call_made 1. Schell, J.; Casas, N.; Pini, R.; Mazzotti, M. Adsorption 2012, 18, 49-65
- external page call_made 2. Casas, N.; Schell, J.; Pini, R.; Mazzotti, M. Adsorption 2012, 18, 143-161
- external page call_made 3. Schell, J.; Casas, N.; Blom, R.; Spjelkavik, A.I.; Andersen, A.; Cavka, J.H.; Mazzotti, M. Adsorption 2012, 18, 213-227
- external page call_made 4. Joss, L.; Mazzotti, M. Adsorption 2012, 18, 381-393
- external page call_made 5. Casas, N.; Schell, J.; Blom, R.; Mazzotti, M. Sep. Purif. Technol. 2013, 112, 34-48
- external page call_made 6. Schell, J.; Casas, N.; Marx, D.; Mazzotti, M. Ind. Eng. Chem. Res. 2013, 52, 8311-8322
- external page call_made 7. Marx, D.; Joss, L.; Casas, N.; Schell, J.; Mazzotti, M. Adsorption 2014, 20, 493-510
- external page call_made 8. Hefti, M; Marx, D; Joss, L; Mazzotti, M. Micropor. Mesopo. Mat. 2015, 215, 215-228